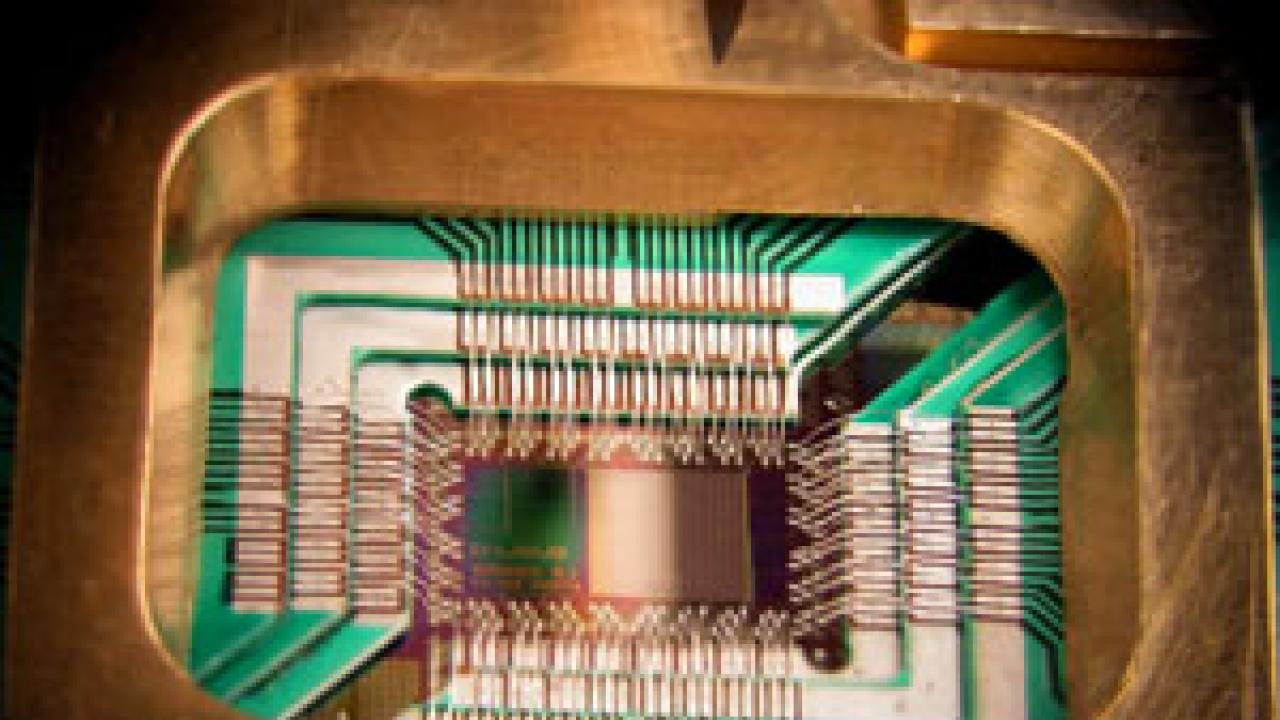
Quantum computing is based on the bizarre world of quantum mechanics, and unsurprisingly this new form of computing offers some bizarre challenges of its own. The physics of the very small follow a drastically different set of rules than your classical apple-falling-out-of-the-tree does, and quantum computing aims to harness these differences to create a powerful new type of computer that could, among other things, help physicists perform particle simulations that are nearly impossible to do on a traditional computer.
"Quantum mechanics has different rules, and now quantum computing emerged because people realized they can use these quantum rules to do computations," says Rosario Fazio, a senior ICTP physicist and head of the Centre’s Condensed Matter and Statistical Physics research section. Fazio, along with several other ICTP scientists and students, study quantum computing and its related fields, tackling various aspects from quantum thermodynamics to figuring out how to know if a quantum computer is actually working. Besides the challenge of building it, a fully-functioning quantum computer has the potential to become a game-changing research tool.
The huge potential for quantum computers lies in the basics of how they operate. Conventional, or classical, computers are built out of miniscule transistors, each capable of storing a "bit" of information. An 'off' transistor corresponds to a 0 and an on transistor to a 1, which combine into long series to produce your desktop experience. Quantum computers rely instead on "qubits," which, thanks to the superposition principle of quantum mechanics, can store a 1, a 0, or the potential for either at the same time, possibly creating the basis for an exponentially more efficient computer.
Although a fully working quantum computer is still a fantasy, the idea excites a lot of physicists who see it as a useful tool for studying the quantum underpinnings of their fields, one that is far more useful than any current classical computer. For example, because classical computers are based on classical physics, if you want to model how ten--just ten--particles interact and behave as a system, "you have to include 2 to the 10 degrees of freedom, representing all the possible configurations of these particles," explains ICTP physicist Antonello Scardicchio. All these degrees of freedom add up to a lot of variables that slow down calculations. "Realistically on a classical computer you can fully simulate the dynamics of only 20 qubits," says Sebastiano Pilati, another ICTP physicist.
With a quantum computer, says Scardicchio, "you could really see how you develop macroscopic physics from microscopic physics, how you get statistical mechanics, thermodynamics, and so on. You can really see the whole movie from three particles, four particles, to four hundred, four thousand particles. This would be great."
But before seeing that full movie, quite a few practical considerations need attention. "Once you have an idea of what a quantum computer should be, then you have to find out how to realize it,” says Fazio. These days, his interests include quantum thermodynamics and quantum information's various connections with the complex systems that statistical physicists have been studying for years. These many-body problems revolve around the repercussions of quantum particles' interactions with each other, known as entanglement. "Entanglement should make a quantum computer more efficient, but this is not completely proven," says Fazio. Recent papers, he explains, suggest that there can be too much entanglement. This conundrum makes for fertile research field.
Besides building them, one of the main challenges surrounding quantum computers is determining what questions a quantum computer could help solve. Scardicchio started work on this question by studying spin glasses, or magnets made of disordered atoms, atoms whose magnetic spin directions are all over the place instead of aligned in a regular pattern. "Eventually, I realized that the physics of quantum spin glasses is actually connected with the other question, which is how you build a quantum computer." Scardicchio has recently started a collaboration with Google, who are also interested in how the study of spin glasses could help resolve the tricky quantum computer problems of environmental interaction and maintaining coherence, or keeping qubits from falling out of their superpositions--losing their ability to hold multiple values at once --when they are disturbed.
The question of how to maintain coherence is also investigated by physicists, like ICTP Postdoctoral fellow John Goold, who study the interface between quantum information and thermodynamics. "I'm interested in describing the thermodynamics of very small systems," Goold explains. Classical thermodynamics is fairly simple: work can be converted into heat and vice versa. This places fundamental limitations on the efficiency of machines. As miniaturization pushes machines, including computers, to become smaller and smaller, quantum properties come into play, and it's not very clear what effects that will have. "When you have quantum entanglement, quantum superposition, what's the thermodynamic repercussion for having such phenomenon that are not present in classical devices?" Goold wonders. Fazio is also interested in these questions, all leading back to his main one: "Will a machine behave differently, just because the internal dynamics are quantum?" The D-Wave quantum computer, developed by a Canadian company to solve only optimization problems and recently purchased by Google, tries to work around this question by operating at very cold temperatures, near 0.02 degrees Kelvin. But, cautions Fazio, "how well the D-Wave works is still a matter of debate."
That, says Sebastiano Pilati, is "actually one of the main problems in this field. You might have a quantum computer, but how do you check if it's working properly or not?" Pilati designs algorithms to simulate quantum systems, to be used on powerful conventional computers. Originally his focus was the behavior of ultra-cold atoms, a system where quantum properties are very evident and easier to study. Practical questions of quantum computing, however, also rest on understanding quantum effects, leading Pilati to apply similar algorithms. "If you have a machine built to do something that at least in principle conventional computers are not capable of doing, you don't know what it is supposed to do," explains Pilati. That's where Pilati’s simulations come in. Because fully modeling a quantum system would quickly overwhelm conventional computers, Pilati's algorithms try to get as close as possible through creative programming. Even then, a computer a thousand times as powerful as an office desktop runs for an entire day to work through these advanced simulations. "And that's what you need to get just one number," laughs Pilati. But these simulations might help puzzle out how, and how well, quantum computers can work.
In the spirit of ICTP’s commitment to international cooperation, Fazio and Scardicchio (along with ICTP scientist Markus Müller) are currently directing a School on Quantum Information Processing with Nanyang Technological University in Singapore. The two week school is gathering scientists and students to discuss how the manipulation, storage, and protection of information can depend on quantum effects. One major discussion, of course, will cover the future and potential of quantum computing, a future in which ICTP physicists and others see great potential for a deeper understanding of our quantum universe.
--Kelsey Calhoun